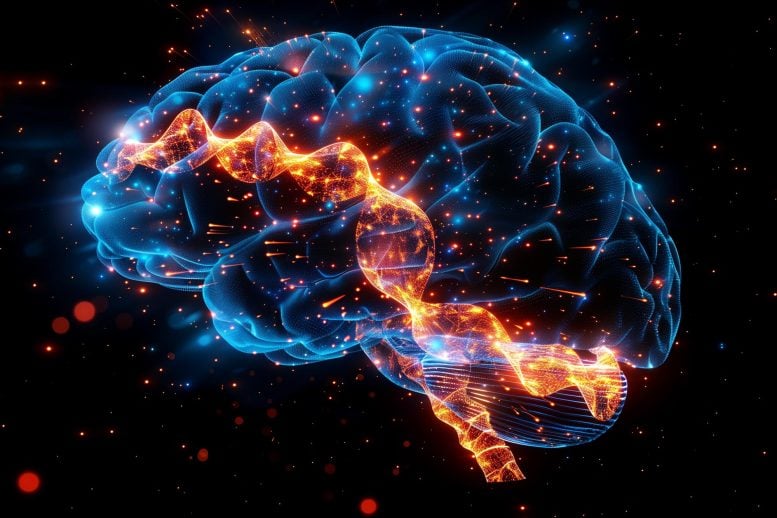
Researchers have developed a revolutionary magnetogenetics technology that can control brain circuits non-invasively using magnetic fields.
This new method, which has been successfully tested in mice, offers promise for treating neurological and psychiatric disorders by allowing precise activation or inhibition of neurons without the need for invasive procedures.
Magnetogenetics and Brain Control
A new non-invasive technology enables the control of specific brain circuits using magnetic fields, according to a preclinical study from researchers at Weill Cornell Medicine, The Rockefeller University, and the Icahn School of Medicine at Mount Sinai. This technology shows great potential as a tool for brain research and as a foundation for future treatments of diverse neurological and psychiatric conditions, including Parkinson’s disease, depression, obesity, and complex pain.
The new gene therapy technology is described in a paper published on October 9 in the journal “We envision that magnetogenetics technology may someday be used to benefit patients in a wide range of clinical settings,” said study senior author Dr. Michael Kaplitt, professor and executive vice-chairman of neurological surgery at Weill Cornell Medicine and director of Movement Disorders Surgery at NewYork-Presbyterian/Weill Cornell Medical Center. The study was a collaboration between Dr. Kaplitt’s laboratory and the laboratories of Dr. Jeffrey Friedman, the Marilyn M. Simpson Professor in the Laboratory of Molecular Genetics at The Rockefeller University; and Dr. Sarah Stanley, an assistant professor in the Department of Medicine at the Icahn School of Medicine at Mt. Sinai. The study’s first author was Dr. Santiago Unda, a postdoctoral researcher in Dr. Kaplitt’s laboratory. Controlling brain circuits in real-time, in a way that allows animals—or humans—to move around normally, has been a major goal for neuroscientists, but a very challenging one. In the laboratory, optogenetics technology, for example, can make selected neurons switch on or off immediately with light pulses, but requires an invasive apparatus for delivering those light pulses to the brain. In the clinic, deep brain stimulation permits modulation of brain regions, but this also requires a permanently implanted device and greater precision also remains a goal. After doing early work on magnetogenetic technology as an alternative to other approaches, Dr. Friedman and Dr. Stanley joined forces with Dr. Kaplitt, a pioneer of brain-targeted gene therapies, to develop a method of this type with the potential for clinical applications. The resulting approach uses gene therapy techniques to deliver an engineered ion-channel protein to a desired type of neuron. The ion channel protein essentially works as a switch to turn affected neurons on or off, and is sensitive to a magnetic field because it includes an antibody-like protein that sticks to a natural iron-trapping protein called ferritin. While the gene therapy is delivered to precise brain regions through a minimally invasive surgery, a sufficiently strong magnetic field can then exert enough force on the ferritin-trapped iron atoms to open or close the channel—activating the neuron or inhibiting it, depending on the design, without the need for an implanted device or drug. In one proof of concept, the team injected the gene therapy for the magnetically sensitive channels into specific neurons within a movement-controlling region called the striatum in mice; they then used the magnetic field from a magnetic resonance imaging machine to activate the neurons and markedly slow, even freeze, the mice’s movements. In another experiment, they reduced neuronal activity in a brain region called the subthalamic nucleus to ameliorate movement abnormalities in a Parkinsonism mouse model. The researchers showed that their method can work even when using a much smaller and less expensive “transcranial magnetic stimulation” device, which is often used currently in the clinic to treat patients with depression, migraine, and other conditions. The experiments uncovered no safety issues, and the researchers noted that normal ambient magnetic fields would be far too weak to trigger magnetogenetic switches inadvertently. The team now intends to explore potential clinical applications including treatments for psychiatric disorders and even chronic pain in peripheral nerves. They also will continue to explore and optimize the magnetogenetics technology itself. “Being able now to do directional manipulations of brain activity with this relatively simple system is going to be very important in helping us better understand the underlying principles to help further advance this new technology,” Dr. Unda said. Reference: “Bidirectional regulation of motor circuits using magnetogenetic gene therapy” by Santiago R. Unda, Lisa E. Pomeranz, Roberta Marongiu, Xiaofei Yu, Leah Kelly, Gholamreza Hassanzadeh, Henrik Molina, George Vaisey, Putianqi Wang, Jonathan P. Dyke, Edward K. Fung, Logan Grosenick, Rick Zirkel, Aldana M. Antoniazzi, Sofya Norman, Conor M. Liston, Chris Schaffer, Nozomi Nishimura, Sarah A. Stanley, Jeffrey M. Friedman and Michael G. Kaplitt, 9 October 2024, Science Advances. Many Weill Cornell Medicine physicians and scientists maintain relationships and collaborate with external organizations to foster scientific innovation and provide expert guidance. The institution makes these disclosures public to ensure transparency. This work was supported by the National Institute of Neurological Disorders and Stroke and the NIH Office of the Director, both part of the Adblock test (Why?)Non-Invasive Techniques for Neural Modulation
Proof of Concept and Future Prospects
DOI: 10.1126/sciadv.adp9150